Difference between revisions of "Examples of Communication Systems/General Description of ISDN"
(20 intermediate revisions by 2 users not shown) | |||
Line 10: | Line 10: | ||
$\rm I$ntegrated $\rm S$ervices $\rm D$igital $\rm N$etwork $\rm (ISDN)$ was the first unified digital network for voice, text, data, images, video and multimedia communications. It was introduced in the late 1980s and and had about 25 million users in Germany in 2004, for example. | $\rm I$ntegrated $\rm S$ervices $\rm D$igital $\rm N$etwork $\rm (ISDN)$ was the first unified digital network for voice, text, data, images, video and multimedia communications. It was introduced in the late 1980s and and had about 25 million users in Germany in 2004, for example. | ||
− | Compared with the analog telecommunications | + | Compared with the analog telecommunications methods that were common before, ISDN has |
*offered many new or enhanced services, | *offered many new or enhanced services, | ||
Line 53: | Line 53: | ||
A distinction is made with ISDN between | A distinction is made with ISDN between | ||
− | *the [[Examples_of_Communication_Systems/ISDN_Basic_Access|"ISDN basic access"]] with two so-called $\text{B channels}$ ("bearer channels") with $\text{64 kbit/s}$ each and one $\text{D channel}$ ("data | + | *the [[Examples_of_Communication_Systems/ISDN_Basic_Access|"ISDN basic access"]] with two so-called $\text{B channels}$ ("bearer channels") with $\text{64 kbit/s}$ each and one $\text{D channel}$ ("data channel") with $\text{16 kbit/s}$, |
*the [[Examples_of_Communication_Systems/ISDN_Primary_Multiplex_Connection|"ISDN primary multiplex connection"]] with thirty "B channels" and one signaling and one synchronization channel, each at $\text{64 kbit/s}$. | *the [[Examples_of_Communication_Systems/ISDN_Primary_Multiplex_Connection|"ISDN primary multiplex connection"]] with thirty "B channels" and one signaling and one synchronization channel, each at $\text{64 kbit/s}$. | ||
Line 67: | Line 67: | ||
<br> | <br> | ||
The available ISDN services can be divided into two groups: | The available ISDN services can be divided into two groups: | ||
− | + | ||
− | '''Bearer services''' are used to transport information and ensure data transmission and switching between the access interfaces of the network. This corresponds to definitions in the first three layers of the [https://en.wikipedia.org/wiki/OSI_model "OSI reference model"]: | + | ⇒ '''Bearer services''' are used to transport information and ensure data transmission and switching between the access interfaces of the network. This corresponds to definitions in the first three layers of the [https://en.wikipedia.org/wiki/OSI_model "OSI reference model"]: |
− | + | [[File:EN_Bei_T_1_1_S2.png|right|frame|Bearer services and tele services with ISDN]] | |
− | + | # $\rm PL$ (Physical Layer), | |
− | + | # $\rm DL$ (Data Link Layer), and | |
+ | # $\rm NL$ (Network Layer). | ||
Bearer services include | Bearer services include | ||
− | *'''circuit-switched services''', for example | + | *'''circuit-switched services''', for example |
− | + | **data transmission with 64 kbit/s $($on the S0 bus or via the X21 terminal adapter$)$ and | |
+ | **audio transmission $($voice and music up to 3400 Hz$)$ as in the analog telephone network, | ||
+ | |||
+ | *'''packet-switched''' services – for example, | ||
+ | **access to the packet network in the "B channel". | ||
+ | |||
− | |||
+ | ⇒ '''Tele services''' are "end-to-end services". They include | ||
+ | *the terminal equipment, | ||
+ | * switching functions and protocols in layers '''1''' to '''3''', and | ||
− | + | * functions for controlling communication processes in layers '''4''' to '''7''' of the OSI reference model. | |
− | |||
− | * functions for controlling communication processes in layers 4 to 7 of the OSI reference model. | ||
The most important tele services are: | The most important tele services are: | ||
− | + | # ISDN telephony with a bandwidth of 3.1 kHz or 7 kHz $($with B–ISDN$)$ – also with transitions to the analog fixed network and to radio networks, | |
− | + | # ISDN teletext with 64 kbit/s and transitions to telebox services $($mailbox$)$, T-Online services and Datex L/P services, | |
− | + | # ISDN telefax, e.g. group 4 telecopier with transitions to group 3, | |
− | + | # ISDN mixed mode, which is understood to mean mixed simultaneous data transmission of texts and images, | |
− | + | # ISDN T–Online with 64 kbit/s and transitions to T-Online in the analog telephone network as well as to group 3 and 4 telefax, | |
− | + | # video telephony – in practice, however, only possible as slow moving image transmission, | |
− | + | # data communications using standardized protocols, such as file transfer with FTAM $($comparable, but technically not identical to the Internet service "FTP"$)$. | |
{{BlaueBox|TEXT= | {{BlaueBox|TEXT= | ||
$\text{Conclusion:}$ | $\text{Conclusion:}$ | ||
− | '''Service features''' as subsets of a service can be divided into three categories: | + | The "'''Service features'''" as subsets of a service can be divided into three categories: |
− | + | :*"'''Access service features'''": Dial-up or fixed connection, line or packet switching, and terminal selection on the S0 bus, | |
− | + | ||
− | + | :*"'''Connection service features'''": Fast connection setup or conference connection, | |
+ | |||
+ | :*'''"Information service feature'''": Event information, identification of other subscribers, display of charges and tariffs.}} | ||
==Network infrastructure for ISDN== | ==Network infrastructure for ISDN== | ||
<br> | <br> | ||
− | ISDN, which was developed in the early 1980s, was intended to use the existing analog telephone network for cost reasons. | + | ISDN, which was developed in the early 1980s, was intended to use the existing analog telephone network for cost reasons. |
− | + | The greatest cost factor of the entire infrastructure is | |
+ | *the subscriber line area between the local exchange $\rm (LE)$ or a main distribution frame $($e.g. "switching office"$)$, and | ||
+ | *the subscribers, as the network branches out to the maximum in this area. | ||
+ | |||
+ | [[File:EN_LZI_T_4_3_S2_neu.png| right|frame|Structure of the local loop area]] | ||
+ | [[File:EN_LZI_T_4_3_S1b.png|right|frame| Bundling and twisting of copper wires]] | ||
− | |||
− | |||
− | + | In Germany, this so-called "last mile" is shorter than $4$ kilometers on average, and in urban areas $90\%$ of the time it is even shorter than $2.8$ kilometers. | |
− | |||
− | |||
+ | Due to the topological conditions, the telephone network is increasingly branching out in a star configuration toward the end customer. | ||
+ | In order to avoid having to lay a separate copper cable to the local exchange for each subscriber, splitters have been installed in between and the lines bundled in correspondingly large cables. | ||
+ | The '''local loop area''' is therefore usually made up as follows: | ||
+ | # The "main cable" with up to $2000$ pairs between the local exchange (or the switching office) frame and a cable branch, | ||
+ | # the "branch cable" between the cable branch and the final branch, with up to $300$ pairs and a maximum length of 500 meters, which is significantly shorter than a main cable, | ||
+ | # the "house connection cable" between the terminal box and the network termination box at the subscriber with two pairs of wires. | ||
− | + | <br><br> | |
− | + | In order to reduce the inductive and capacitive influences of neighboring pairs of conductors and thus increase the packing density, two pairs of twisted pairs are twisted into a so-called "star quad". | |
− | In order to reduce the inductive and capacitive influences of neighboring pairs of conductors and thus increase the packing density, two pairs of twisted pairs are twisted into a so-called | ||
+ | The figure shows such a star quad and a bundle cable. In the example shown: | ||
+ | *Five such quads each are combined to form a basic bundle. | ||
− | + | *Five basic bundles are combined into one main bundle. | |
− | |||
− | * | ||
− | + | *The cable contains $50$ pairs with PE insulation. | |
+ | <br clear=all> | ||
==Attenuation behavior of copper cables== | ==Attenuation behavior of copper cables== | ||
<br> | <br> | ||
− | In the past, copper two-wire lines | + | In the past, copper two-wire lines – usually designated "Cu" in network diagrams – with core diameters of $0.35$ mm, $0.4$ mm and $0.5$ mm were laid in the area of the "Deutsche Bundespost" (today: "Deutsche Telekom"). |
− | + | [[File:P_ID1490__Bei_T_1_1_S3c_v1.png|right|frame|Attenuation curve of a $0.4$ mm two-wire line]] | |
− | |||
+ | *In the chapter "Properties of Electrical Cables" of the book [[Lineare zeitinvariante Systeme|"Linear and Time Invariant Systems"]], the electrical properties of copper lines are described in detail. | ||
− | + | *Here we limit ourselves to a few properties which are of interest with regard to their use with ISDN. | |
− | |||
− | For these, for example, in [PW95]<ref name='PW95'>Pollakowski, M.; Wellhausen, H.W.: | + | |
+ | All following statements refer to lines with $0.4$ mm diameter. For these, for example, in [PW95]<ref name='PW95'>Pollakowski, M.; Wellhausen, H.W.: Eigenschaften symmetrischer Ortsanschlusskabel im Frequenzbereich bis 30 MHz. Mitteilung aus dem Forschungs- und Technologiezentrum der Deutschen Telekom AG, Darmstadt, Verlag für Wissenschaft und Leben Georg Heidecker, 1995.</ref> the following empirically found attenuation and phase curve were given, where $l$ denotes the cable length: | ||
:$${a_{\rm K}(f)}/{ {\rm dB}} = \left [ 5.1 + 14.3 \cdot \left ({f}/{ {\rm MHz}}\right )^{0.59}\right ]\cdot{l}/{ {\rm km}} | :$${a_{\rm K}(f)}/{ {\rm dB}} = \left [ 5.1 + 14.3 \cdot \left ({f}/{ {\rm MHz}}\right )^{0.59}\right ]\cdot{l}/{ {\rm km}} | ||
Line 150: | Line 165: | ||
\hspace{0.05cm}.$$ | \hspace{0.05cm}.$$ | ||
− | With the interactive applet [[Applets: | + | With the interactive HTML5/JavaScript applet [[Applets:Attenuation_of_Copper_Cables|"Attenuation of copper cables"]] you can view the attenuation curve of symmetrical lines and coaxial cables with different dimensions and with the (German language) SWF applet the [[Applets:Zeitverhalten_von_Kupferkabeln|"Time behavior of copper cables"]]. |
− | + | ||
The diagram shows the attenuation curve in the frequency range up to $1 \ \rm MHz$ for the cable lengths $l = 1 \ \rm km$, $l = 2 \ \rm km$ and $l = 4 \ \rm km$ with a cable diameter of $\text{0.4 mm}$. One can see from this diagram: | The diagram shows the attenuation curve in the frequency range up to $1 \ \rm MHz$ for the cable lengths $l = 1 \ \rm km$, $l = 2 \ \rm km$ and $l = 4 \ \rm km$ with a cable diameter of $\text{0.4 mm}$. One can see from this diagram: | ||
− | + | # The attenuation function $a_{\rm K}(f)$ for one kilometer of cable length is between $5.1 \ \rm dB$ $($at $f = 0)$ and $19.4 \ \rm dB$ $($at $f = 1 \ \rm MHz)$ and is proportional to the cable length. For $l = 4 \ \rm km$, these values quadruple. | |
− | + | # Cables with a length of $4 \ \rm km$ occur in ISDN at most on the $\rm U_{\rm K0}$ bus, i.e. on the connection between the local exchange and the terminal. Due to the 4B3T coding, the symbol sequence frequency here is only $120 \ \rm kHz$. | |
− | + | # In the diagram, this ISDN–relevant range is highlighted in yellow. At $f = 120 \ \rm kHz$ and $l = 4 \ \rm km$, the attenuation is approx. $37 \ \rm dB$, which is rather moderate compared to broadband [[Examples_of_Communication_Systems/General_Description_of_DSL#.23_OVERVIEW_OF_THE_SECOND_MAIN_CHAPTER_.23|"DSL"]] ("'Digital Subscriber Line"). This means: Cable attenuation is not critical for ISDN. | |
− | + | # The above attenuation curve only applies to the "two-wire line" transmission medium. In the ISDN access network, however, there are also transformers with the consequence that DC signal components cannot be transmitted over them. | |
− | + | # For the ISDN system, this fact means that in the access network $($on the $\rm U_{\rm K0}$ bus$)$, line coding – more precisely, the [[Digital_Signal_Transmission/Block_Coding_with_4B3T_Codes|"4B3T code"]] – must be used to ensure that the transmitted signal is free of DC signals. | |
− | + | # Furthermore, it must be taken into account that in two-wire lines in cable bundles, the [[Linear_and_Time_Invariant_Systems/Properties_of_Balanced_Copper_Pairs#Crosstalk_on_two-wire_lines|"crosstalk from neighboring wires"]] is the dominant source of noise and not, for example, the thermal noise as in a coaxial cable system. | |
− | + | # Therefore, the bit error probability cannot be reduced here by increasing the transmitting power, since a higher level would amplify the noise signal $($for other pairs$)$ in the same way as the useful signal. | |
− | + | # Of the crosstalk noise, "'''near-end crosstalk'''" is more critical than "far-end crosstalk". Near-end crosstalk occurs when two adjacent pairs of wires are operated in different directions, so that the disturbed receiver is located close to the interfering transmitter. | |
+ | # In "far-end crosstalk", on the other hand, the induced noise power is noticeably attenuated by the cable attenuation and thus has less disturbing influence. | ||
==Four-wire and two-wire transmission== | ==Four-wire and two-wire transmission== | ||
<br> | <br> | ||
− | A communication link usually operates – as is the case with ISDN – in '''full duplex mode''', i.e. the two communication partners transmit continuously and independently of each other. | + | A communication link usually operates – as is the case with ISDN – in '''full duplex mode''', i.e. the two communication partners transmit continuously and independently of each other. |
− | + | [[File:EN_Bei_T_1_1_S4neu.png|right|frame|Four-wire and two-wire transmission]] | |
− | |||
− | |||
− | + | *To ensure this mode of operation, different variants are possible, which are shown in the diagram on the right. | |
− | + | *The transmitting and receiving device at the customer $($subscriber $\rm A)$ is called "Network Termination" $\rm (NT)$. | |
− | + | ||
− | + | *The corresponding remote station in the local exchange is called "Line Termination" $\rm (LT)$. | |
+ | |||
+ | |||
+ | There are two possibilities for such full duplex operation: | ||
+ | |||
+ | '''(1)''': Communication from $\rm A$ → $\rm B$ and the opposite direction from $\rm B$ → $\rm A$ can be implemented via separate lines. | ||
+ | |||
+ | :Such "'''four-wire transmission'''" is used with ISDN in the house connection area – the so-called $\rm S_{\rm 0}$ bus – where one twisted pair is provided for each direction. | ||
+ | |||
+ | '''(2)''': More economical is the shared use of a twisted pair for both directions – i.e., so-called "'''two-wire transmission'''". | ||
+ | :This is used for ISDN in the access network – on the $\rm U_{\rm K0}$ bus. Since the same frequency range is used for both directions, this is also referred to as "two-wire frequency rectification". | ||
<br clear=all> | <br clear=all> | ||
{{BlaueBox|TEXT= | {{BlaueBox|TEXT= | ||
− | $\text{ | + | $\text{Conclusions:}$ |
− | *In '''four-wire transmission''', | + | *In '''four-wire transmission''', near-end crosstalk (see previous section) can occur over the first few meters of the line due to inductive or capacitive couplings, i.e. the transmitter interferes with its own receiver. |
+ | |||
+ | *In the '''two-wire variant''', the internal reflection of the transmitted signal into the (own) receiver is the dominant cause of noise, which can be avoided or reduced for narrowband transmitted signals (for example, speech) by means of a [[Examples_of_Communication_Systems/ISDN_Basic_Access#Directional_separation_method|"fork circuit"]]. | ||
− | * | + | *For wideband signals, '''additional complex adaptive methods for echo cancellation''' are required.}} |
+ | ==Some basics of pulse code modulation== | ||
+ | <br> | ||
+ | The ISDN concept is largely based on '''Pulse code modulation''' $\rm (PCM)$, the basic features of which were developed as early as 1938 by [https://en.wikipedia.org/wiki/Alec_Reeves Alec Reeves]. This important basic field for digital modulation and digital signal transmission is described in detail in the book [[Modulation_Methods/Pulse_Code_Modulation|"Modulation Methods"]]. | ||
− | + | [[File:EN_Mod_T_4_1_S1_v2.png|right|frame|Principle of Pulse Code Modulation $\rm (PCM)$ | |
− | <br> | + | |
− | + | $q(t)\ \circ\!\!-\!\!\!-\!\!\!-\!\!\bullet\,\ Q(f)$ ⇒ source signal (from German: "Quellensignal"), analog<br> | |
+ | $q_{\rm A}(t)\ \circ\!\!-\!\!\!-\!\!\!-\!\!\bullet\,\ Q_{\rm A}(f)$ ⇒ sampled source signal (from German: "abgetastet" ⇒ "A")<br> | ||
+ | $q_{\rm Q}(t)\ \circ\!\!-\!\!\!-\!\!\!-\!\!\bullet\,\ Q_{\rm Q}(f)$ ⇒ quantized source signal (from German: "quantisiert" ⇒ "Q")<br> | ||
+ | $q_{\rm C}(t)\ \circ\!\!-\!\!\!-\!\!\!-\!\!\bullet\,\ Q_{\rm C}(f)$ ⇒ coded source signal (from German: "codiert" ⇒ "C"), binary <br> | ||
+ | $s(t)\ \circ\!\!-\!\!\!-\!\!\!-\!\!\bullet\,\ S(f)$ ⇒ transmitted signal (from German: "Sendesignal"), digital<br> | ||
+ | $n(t)$ ⇒ noise signal, characterized by the power-spectral density ${\it Φ}_n(f)$, analog | ||
+ | $r(t)= s(t) \star h_{\rm K}(t) + n(t)$ ⇒ received signal, $h_{\rm K}(t)\ \circ\!\!-\!\!\!-\!\!\!-\!\!\bullet\,\ H_{\rm K}(f)$, analog<br> | ||
+ | Note: Spectrum $R(f)$ can not be specified due to the stochastic component $n(t)$.<br> | ||
+ | $v_{\rm C}(t)\ \circ\!\!-\!\!\!-\!\!\!-\!\!\bullet\,\ V_{\rm C}(f)$ ⇒ signal after decision, binary<br> | ||
+ | $v_{\rm Q}(t)\ \circ\!\!-\!\!\!-\!\!\!-\!\!\bullet\,\ V_{\rm Q}(f)$ ⇒ signal after PCM decoding, $M$–level<br> | ||
+ | Note: On the receiver side, there is no counterpart to "Quantization"<br> | ||
+ | $v(t)\ \circ\!\!-\!\!\!-\!\!\!-\!\!\bullet\,\ V(f)$ ⇒ sink signal, analog<br>]] | ||
+ | |||
+ | Here follows a short summary with regard to the use with ISDN. The graphic shows the block diagram of the PCM transmission system, which is adapted to the situation with ISDN. You can see: | ||
+ | |||
+ | *The analog (i.e.: value and time continuous) source signal $q(t)$ is converted into the binary signal $q_{\rm C}(t)$ by the three function blocks '''Sampling – Quantization – PCM Coding'''. In the graphic, this is shown in the upper signal path. | ||
+ | |||
+ | *The block with gray background shows the "digital transmission system" with transmitter, channel distortions and noise addition and the receiver, which among other things contains a decision. The channel output signal $v_{\rm C}(t)$ is binary like $q_{\rm C}(t)$. | ||
+ | |||
+ | *In the lower branch you can see the PCM decoder with the time-discrete, but now higher-level output signal $v_{\rm Q}(t)$. This is followed by the '''signal reconstruction''' to obtain the analog signal $v(t)$, for which an ideal rectangular low-pass filter is sufficient. | ||
− | + | *There is no equivalent for the quantization at the receiver end. This means: The quantization errors that are unavoidable at the transmitter are irreversible. For this reason, $v(t) ≠ q(t)$ applies in principle to PCM as to any form of digital signal transmission. | |
− | + | *An important quantization parameter is the level number $M = 2^N$, where $N$ indicates the number of binary characters required for a sample. | |
− | |||
− | |||
− | |||
− | |||
− | *An important quantization parameter is the number | ||
+ | *The larger $N$, the less the interfering influence of quantization and the higher the quality of the PCM system. | ||
− | |||
+ | All these statements apply to PCM in general. Now the special features of pulse code modulation at ISDN are mentioned. | ||
+ | <br clear=all> | ||
{{GraueBox|TEXT= | {{GraueBox|TEXT= | ||
− | $\text{Pulse code modulation with ISDN:}$ The sampling in the time interval $T_{\rm A}$ takes place according to the [[Signal_Representation/Discrete-Time_Signal_Representation#Sampling_theorem|"sampling theorem"]]. This states: | + | $\text{Pulse code modulation with ISDN:}$ |
− | *If the spectrum $Q(f)$ of the analog source signal has components up to the frequency $f_{\rm NF, \hspace{0. | + | |
+ | The sampling in the time interval $T_{\rm A}$ takes place according to the [[Signal_Representation/Discrete-Time_Signal_Representation#Sampling_theorem|"sampling theorem"]]. This states: | ||
+ | *If the spectrum $Q(f)$ of the analog source signal $q(t)$ has components up to the frequency $f_{\rm NF, \hspace{0.1cm}max}$, the following must apply for the sampling rate: | ||
:$$f_{\rm A} = \frac{1}{T_{\rm A} } \ge 2 \cdot f_{\rm NF, \hspace{0.05cm}max} | :$$f_{\rm A} = \frac{1}{T_{\rm A} } \ge 2 \cdot f_{\rm NF, \hspace{0.05cm}max} | ||
\hspace{0.05cm}.$$ | \hspace{0.05cm}.$$ | ||
− | *ISDN telephone signals contain spectral components between $300 \ \rm Hz$ and $3400 \ \rm Hz$ and the sampling rate is $f_{\rm A} = 8 \ \rm kHz$ ⇒ $T_{\rm A} = 125 \ \rm µ s$. Thus, the sampling theorem is satisfied. | + | *ISDN telephone signals contain spectral components between $300 \ \rm Hz$ and $3400 \ \rm Hz$ and the sampling rate is $f_{\rm A} = 8 \ \rm kHz$ ⇒ $T_{\rm A} = 125 \ \rm µ s$. Thus, the sampling theorem is satisfied. |
− | As mentioned before, quantization to $M$ possible signal values leads to irreversible errors. Because of the following binary PCM coding, a power of two is always chosen for $M$. Thus, each of the $M$–level input values can be represented by $N = \log_2 \hspace{0.05cm} (M)$ binary symbols (bits). | + | ⇒ As mentioned before, quantization to $M$ possible signal values leads to irreversible errors. Because of the following binary PCM coding, a power of two is always chosen for $M$. Thus, each of the $M$–level input values can be represented by $N = \log_2 \hspace{0.05cm} (M)$ binary symbols ("bits"). In this dimensioning, it should be noted: |
+ | *The "quantization signal-to-noise power ratio" is $ρ_{\rm Q} ≈ M^2 = 2^{2N}$. This quantity describes the resulting SNR $ρ_v$ at the sink, assuming no additional transmission errors. When interference $($or noise$)$ is taken into account, the sink SNR $ρ_v$ is always smaller than the quantization SNR $ρ_{\rm Q}$. | ||
− | + | *By using large values of $M$ or $N$, one can increase the PCM quality at the expense of the effort, the transmission rate, and the high-fruquency bandwidth thus required. For ISDN, a good compromise $($for the 1990s$)$ between desirable quality and required bit rate was standardized with $N = 8$ ⇒ $M = 256$. | |
− | + | ||
− | *By using large values of $M$ or $N$, one can increase the PCM quality at the expense of the effort, the transmission rate, and the | + | *The ISDN bit rate $($for each of the two bearer channels$)$ is $8 · 8000 \ \rm 1/s = 64 \ kbit/s$ according to the above specifications. The quantization SNR is thus |
− | *The ISDN bit rate (for each of the two | ||
:$$\rho_{\rm Q} = 2^{16}\hspace{0.3cm}\Rightarrow \hspace{0.3cm} | :$$\rho_{\rm Q} = 2^{16}\hspace{0.3cm}\Rightarrow \hspace{0.3cm} | ||
Line 221: | Line 268: | ||
\hspace{0.05cm}.$$ | \hspace{0.05cm}.$$ | ||
− | *CD quality $(N = 16 \ ⇒ \ M = | + | *Compact disc $\rm (CD)$ quality $(N = 16 \ ⇒ \ M = 65\hspace{0.05cm}536)$ would result in $10 · \lg ρ_{\rm Q} ≈ 96 \ \rm dB$. However, this would require doubling the bit rate to $128 \ \rm kbit/s$. |
− | Let us now look at the gray block in the PCM block diagram. In ISDN, the transmitter does not include a modulator for frequency conversion and the receiver does not include a demodulator. In other words: ISDN is a '''baseband transmission system''' with the following special features: | + | Let us now look at the gray block in the PCM block diagram. In ISDN, the transmitter does not include a modulator for frequency conversion and the receiver does not include a demodulator. In other words: ISDN is a '''baseband transmission system''' with the following special features: |
− | + | # In the ISDN transmission system, a redundant ternary transmitted signal $s(t)$ is used, with the "modified AMI code" on the $S_0$ interface $($"house connection"$)$ and "4B3T coding" on the $U_{\rm K0}$ interface $($"access network"$)$. | |
− | + | # The dominant noise $n(t)$ is the "near-end crosstalk" of adjacent line pairs. Many of the statements given in the book [[Digital_Signal_Transmission/System_Components_of_a_Baseband_Transmission_System#Transmission_channel_and_interference|"Digital Signal Transmission"]] for AWGN noise are only conditionally valid for this type of noise.}} | |
==Origin and historical development== | ==Origin and historical development== | ||
<br> | <br> | ||
− | In the following, some data on the historical development of digital transmission and switching technology – especially ISDN – are compiled. Here we restrict ourselves predominantly to the developments in Germany. Further information can be found in [Sie02]<ref>Siegmund, G.: | + | In the following, some data on the historical development of digital transmission and switching technology – especially ISDN – are compiled. Here we restrict ourselves predominantly to the developments in Germany. Further information can be found in [Sie02]<ref>Siegmund, G.: Technik der Netze. 5. Auflage. Heidelberg: Hüthig, 2002.</ref>. |
− | *'''Around 1970''' | + | |
− | *'''1979''' Decision by Deutschen Bundespost (DBP) to digitize all exchanges. | + | *'''Around 1970''' The need for digital subscriber lines is recognized worldwide; this is the beginning of digital transmission technology with pulse code modulation. |
− | *'''Around 1980''' First ISDN specification by | + | |
− | *'''1982''' Decision of the DBP for the introduction of ISDN and concretization of the plans. However, it takes another seven years before it is introduced. | + | *'''1979''' Decision by Deutschen Bundespost $\rm (DBP)$ to digitize all exchanges. |
+ | |||
+ | *'''Around 1980''' First ISDN specification by "Comité Consultatif International Téléphonique et Télégraphique" $\rm (CCITT)$ – today "International Telecommunication Union" $\rm (ITU)$. | ||
+ | |||
+ | *'''1982''' Decision of the DBP for the introduction of ISDN and concretization of the plans. However, it takes another seven years before it is introduced. | ||
+ | |||
*'''1984/85''' The DBP puts the first digital long-distance and local exchanges into operation. | *'''1984/85''' The DBP puts the first digital long-distance and local exchanges into operation. | ||
− | *'''1987''' Start of two ISDN pilot projects of the DBP in Mannheim and Stuttgart. | + | |
− | *'''1989''' Official start of operation of national ISDN on March 8 at CeBIT in Hanover; specification of a uniform Europe-wide ISDN ( | + | *'''1987''' Start of two German ISDN pilot projects of the DBP in Mannheim and Stuttgart. |
− | *'''1993/94''' ISDN coverage in the old federal states of the Federal Republic of Germany; start of Deutsche Telekom's broadband ISDN pilot project (ATM). | + | |
− | *'''1995''' Official introduction of pan-European ISDN based on the [https://en.wikipedia.org/wiki/Digital_Subscriber_System_No._1 "DSS1 standard"] ( | + | *'''1989''' Official start of operation of national ISDN on March 8 at CeBIT in Hanover; specification of a uniform Europe-wide ISDN $($"Euro–ISDN"$)$. |
+ | |||
+ | *'''1993/94''' ISDN coverage in the old federal states of the Federal Republic of Germany; start of Deutsche Telekom's broadband ISDN pilot project $\rm (ATM)$. | ||
+ | |||
+ | *'''1995''' Official introduction of pan-European ISDN based on the [https://en.wikipedia.org/wiki/Digital_Subscriber_System_No._1 "DSS1 standard"] $($"Euro–ISDN"$)$. | ||
+ | |||
*'''1996''' Introduction of the broadband ISDN bill service. | *'''1996''' Introduction of the broadband ISDN bill service. | ||
+ | |||
*'''1998''' Fully digitized network in Germany. | *'''1998''' Fully digitized network in Germany. | ||
− | The | + | The left diagram of the following figure shows the increase of ISDN subscribers in Germany (blue bars). |
− | |||
− | |||
− | + | [[File:EN_Bei_T_1_1_S6.png|right|frame|Number of narrowband and broadband lines in Germany around the year 2000]] | |
− | |||
− | + | # As early as 1999, the ten million mark is passed and by 2002, there are already 20 million ISDN subscribers in Germany. | |
− | + | # By 2004, half of all narrowband channels are already digital, after the number of analog telephone lines had already begun to decline significantly in 2000. | |
+ | # However, the graph also shows a certain saturation (in mathematical terms: a negative second derivative) of the ISDN curve. | ||
+ | # This is directly related to the success story of $\rm DSL$ ("Digital Subscriber Line"), which began around 2001. | ||
Latest revision as of 17:31, 16 November 2022
Contents
- 1 # OVERVIEW OF THE FIRST MAIN CHAPTER #
- 2 Goals and features of ISDN
- 3 Services and service features of ISDN
- 4 Network infrastructure for ISDN
- 5 Attenuation behavior of copper cables
- 6 Four-wire and two-wire transmission
- 7 Some basics of pulse code modulation
- 8 Origin and historical development
- 9 Exercises for the chapter
- 10 References
# OVERVIEW OF THE FIRST MAIN CHAPTER #
$\rm I$ntegrated $\rm S$ervices $\rm D$igital $\rm N$etwork $\rm (ISDN)$ was the first unified digital network for voice, text, data, images, video and multimedia communications. It was introduced in the late 1980s and and had about 25 million users in Germany in 2004, for example.
Compared with the analog telecommunications methods that were common before, ISDN has
- offered many new or enhanced services,
- faster transmission,
- better voice quality, and
- an easier use of multiple devices.
The ISDN concept and implementation are presented and explained in this chapter. In particular are covered:
- a »general description« of ISDN,
- the »services and service features« of ISDN,
- the »ISDN network infrastructure« and the »different types of connection«,
- the »logical channels and interfaces« of ISDN,
- the most important »transmission codes« of ISDN, and
- »broadband ISDN« as a further development.
At the time of the English translation of this book $\rm (2022)$, however, it must not be concealed:
- ISDN will no longer have a great future in Germany either - in other countries it never had such great importance.
- The German provider »Deutsche Telekom« had long announced that it would switch off ISDN in 2018 and replace it with its "Next Generation Network" $\rm (NGN)$ with packet-switched network infrastructure.
- Vodafone will follow this example by 2022 at the latest.
⇒ We have nevertheless decided to continue this chapter $($which was highly topical in the early 2000s$)$ in the $\rm LNTwww$. However, clearly shortened. Although it lacks the topicality of the day, it is now more of a technical history contribution.
Goals and features of ISDN
The $\rm ISDN$ ("Integrated Services Digital Network") standard, established since the late 1980s, is a service–integrated digital communications network with the aim,
- to digitize the analog signal transmission over telephone lines that had been common until then, thereby achieving better voice quality,
- to continue using the network infrastructure available for analog signal transmission – in particular the copper cables laid at great expense over many years,
- to integrate different sources of information such as voice, text, data and video, as well as the emerging multimedia communications, into a single network,
- to enable different telecommunication services such as telephoning, faxing, Internet surfing, etc. to be provided simultaneously over the existing network of lines,
- to keep the number of lines required as low as possible without compromising the quality of the transmission, and finally
- to provide a data rate of $\text{64 kbit/s}$, which was also considered sufficient for data traffic when ISDN was introduced.
A distinction is made with ISDN between
- the "ISDN basic access" with two so-called $\text{B channels}$ ("bearer channels") with $\text{64 kbit/s}$ each and one $\text{D channel}$ ("data channel") with $\text{16 kbit/s}$,
- the "ISDN primary multiplex connection" with thirty "B channels" and one signaling and one synchronization channel, each at $\text{64 kbit/s}$.
By bundling two channels, the data rate could be increased to $\text{128 kbit/s}$.
- Since the introduction of ISDN in March 1989, the quality of voice transmission and the bit error rate during data transmission have also been steadily improved.
- The ATM-based "broadband ISDN" $\rm (B–ISDN)$ standardized in 1994 also allows significantly higher data rates.
Services and service features of ISDN
The available ISDN services can be divided into two groups:
⇒ Bearer services are used to transport information and ensure data transmission and switching between the access interfaces of the network. This corresponds to definitions in the first three layers of the "OSI reference model":
- $\rm PL$ (Physical Layer),
- $\rm DL$ (Data Link Layer), and
- $\rm NL$ (Network Layer).
Bearer services include
- circuit-switched services, for example
- data transmission with 64 kbit/s $($on the S0 bus or via the X21 terminal adapter$)$ and
- audio transmission $($voice and music up to 3400 Hz$)$ as in the analog telephone network,
- packet-switched services – for example,
- access to the packet network in the "B channel".
⇒ Tele services are "end-to-end services". They include
- the terminal equipment,
- switching functions and protocols in layers 1 to 3, and
- functions for controlling communication processes in layers 4 to 7 of the OSI reference model.
The most important tele services are:
- ISDN telephony with a bandwidth of 3.1 kHz or 7 kHz $($with B–ISDN$)$ – also with transitions to the analog fixed network and to radio networks,
- ISDN teletext with 64 kbit/s and transitions to telebox services $($mailbox$)$, T-Online services and Datex L/P services,
- ISDN telefax, e.g. group 4 telecopier with transitions to group 3,
- ISDN mixed mode, which is understood to mean mixed simultaneous data transmission of texts and images,
- ISDN T–Online with 64 kbit/s and transitions to T-Online in the analog telephone network as well as to group 3 and 4 telefax,
- video telephony – in practice, however, only possible as slow moving image transmission,
- data communications using standardized protocols, such as file transfer with FTAM $($comparable, but technically not identical to the Internet service "FTP"$)$.
$\text{Conclusion:}$ The "Service features" as subsets of a service can be divided into three categories:
- "Access service features": Dial-up or fixed connection, line or packet switching, and terminal selection on the S0 bus,
- "Connection service features": Fast connection setup or conference connection,
- "Information service feature": Event information, identification of other subscribers, display of charges and tariffs.
Network infrastructure for ISDN
ISDN, which was developed in the early 1980s, was intended to use the existing analog telephone network for cost reasons.
The greatest cost factor of the entire infrastructure is
- the subscriber line area between the local exchange $\rm (LE)$ or a main distribution frame $($e.g. "switching office"$)$, and
- the subscribers, as the network branches out to the maximum in this area.
In Germany, this so-called "last mile" is shorter than $4$ kilometers on average, and in urban areas $90\%$ of the time it is even shorter than $2.8$ kilometers.
Due to the topological conditions, the telephone network is increasingly branching out in a star configuration toward the end customer.
In order to avoid having to lay a separate copper cable to the local exchange for each subscriber, splitters have been installed in between and the lines bundled in correspondingly large cables.
The local loop area is therefore usually made up as follows:
- The "main cable" with up to $2000$ pairs between the local exchange (or the switching office) frame and a cable branch,
- the "branch cable" between the cable branch and the final branch, with up to $300$ pairs and a maximum length of 500 meters, which is significantly shorter than a main cable,
- the "house connection cable" between the terminal box and the network termination box at the subscriber with two pairs of wires.
In order to reduce the inductive and capacitive influences of neighboring pairs of conductors and thus increase the packing density, two pairs of twisted pairs are twisted into a so-called "star quad".
The figure shows such a star quad and a bundle cable. In the example shown:
- Five such quads each are combined to form a basic bundle.
- Five basic bundles are combined into one main bundle.
- The cable contains $50$ pairs with PE insulation.
Attenuation behavior of copper cables
In the past, copper two-wire lines – usually designated "Cu" in network diagrams – with core diameters of $0.35$ mm, $0.4$ mm and $0.5$ mm were laid in the area of the "Deutsche Bundespost" (today: "Deutsche Telekom").
- In the chapter "Properties of Electrical Cables" of the book "Linear and Time Invariant Systems", the electrical properties of copper lines are described in detail.
- Here we limit ourselves to a few properties which are of interest with regard to their use with ISDN.
All following statements refer to lines with $0.4$ mm diameter. For these, for example, in [PW95][1] the following empirically found attenuation and phase curve were given, where $l$ denotes the cable length:
- $${a_{\rm K}(f)}/{ {\rm dB}} = \left [ 5.1 + 14.3 \cdot \left ({f}/{ {\rm MHz}}\right )^{0.59}\right ]\cdot{l}/{ {\rm km}} \hspace{0.05cm},$$
- $${b_{\rm K}(f)}/{ {\rm rad}} = \left [ 32.9 \cdot \frac{f}{ {\rm MHz}} + 2.26 \cdot \left ({f}/{ {\rm MHz}}\right )^{0.5}\right ]\cdot{l}/{ {\rm km}} \hspace{0.05cm}.$$
With the interactive HTML5/JavaScript applet "Attenuation of copper cables" you can view the attenuation curve of symmetrical lines and coaxial cables with different dimensions and with the (German language) SWF applet the "Time behavior of copper cables".
The diagram shows the attenuation curve in the frequency range up to $1 \ \rm MHz$ for the cable lengths $l = 1 \ \rm km$, $l = 2 \ \rm km$ and $l = 4 \ \rm km$ with a cable diameter of $\text{0.4 mm}$. One can see from this diagram:
- The attenuation function $a_{\rm K}(f)$ for one kilometer of cable length is between $5.1 \ \rm dB$ $($at $f = 0)$ and $19.4 \ \rm dB$ $($at $f = 1 \ \rm MHz)$ and is proportional to the cable length. For $l = 4 \ \rm km$, these values quadruple.
- Cables with a length of $4 \ \rm km$ occur in ISDN at most on the $\rm U_{\rm K0}$ bus, i.e. on the connection between the local exchange and the terminal. Due to the 4B3T coding, the symbol sequence frequency here is only $120 \ \rm kHz$.
- In the diagram, this ISDN–relevant range is highlighted in yellow. At $f = 120 \ \rm kHz$ and $l = 4 \ \rm km$, the attenuation is approx. $37 \ \rm dB$, which is rather moderate compared to broadband "DSL" ("'Digital Subscriber Line"). This means: Cable attenuation is not critical for ISDN.
- The above attenuation curve only applies to the "two-wire line" transmission medium. In the ISDN access network, however, there are also transformers with the consequence that DC signal components cannot be transmitted over them.
- For the ISDN system, this fact means that in the access network $($on the $\rm U_{\rm K0}$ bus$)$, line coding – more precisely, the "4B3T code" – must be used to ensure that the transmitted signal is free of DC signals.
- Furthermore, it must be taken into account that in two-wire lines in cable bundles, the "crosstalk from neighboring wires" is the dominant source of noise and not, for example, the thermal noise as in a coaxial cable system.
- Therefore, the bit error probability cannot be reduced here by increasing the transmitting power, since a higher level would amplify the noise signal $($for other pairs$)$ in the same way as the useful signal.
- Of the crosstalk noise, "near-end crosstalk" is more critical than "far-end crosstalk". Near-end crosstalk occurs when two adjacent pairs of wires are operated in different directions, so that the disturbed receiver is located close to the interfering transmitter.
- In "far-end crosstalk", on the other hand, the induced noise power is noticeably attenuated by the cable attenuation and thus has less disturbing influence.
Four-wire and two-wire transmission
A communication link usually operates – as is the case with ISDN – in full duplex mode, i.e. the two communication partners transmit continuously and independently of each other.
- To ensure this mode of operation, different variants are possible, which are shown in the diagram on the right.
- The transmitting and receiving device at the customer $($subscriber $\rm A)$ is called "Network Termination" $\rm (NT)$.
- The corresponding remote station in the local exchange is called "Line Termination" $\rm (LT)$.
There are two possibilities for such full duplex operation:
(1): Communication from $\rm A$ → $\rm B$ and the opposite direction from $\rm B$ → $\rm A$ can be implemented via separate lines.
- Such "four-wire transmission" is used with ISDN in the house connection area – the so-called $\rm S_{\rm 0}$ bus – where one twisted pair is provided for each direction.
(2): More economical is the shared use of a twisted pair for both directions – i.e., so-called "two-wire transmission".
- This is used for ISDN in the access network – on the $\rm U_{\rm K0}$ bus. Since the same frequency range is used for both directions, this is also referred to as "two-wire frequency rectification".
$\text{Conclusions:}$
- In four-wire transmission, near-end crosstalk (see previous section) can occur over the first few meters of the line due to inductive or capacitive couplings, i.e. the transmitter interferes with its own receiver.
- In the two-wire variant, the internal reflection of the transmitted signal into the (own) receiver is the dominant cause of noise, which can be avoided or reduced for narrowband transmitted signals (for example, speech) by means of a "fork circuit".
- For wideband signals, additional complex adaptive methods for echo cancellation are required.
Some basics of pulse code modulation
The ISDN concept is largely based on Pulse code modulation $\rm (PCM)$, the basic features of which were developed as early as 1938 by Alec Reeves. This important basic field for digital modulation and digital signal transmission is described in detail in the book "Modulation Methods".
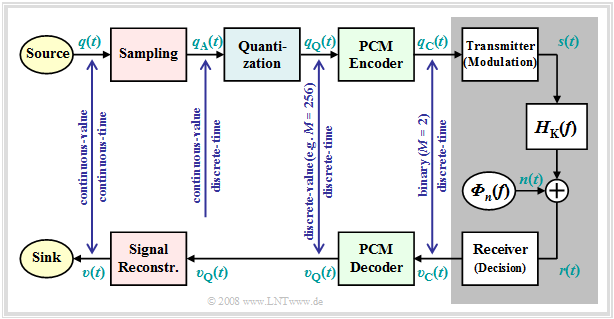
$q_{\rm A}(t)\ \circ\!\!-\!\!\!-\!\!\!-\!\!\bullet\,\ Q_{\rm A}(f)$ ⇒ sampled source signal (from German: "abgetastet" ⇒ "A")
$q_{\rm Q}(t)\ \circ\!\!-\!\!\!-\!\!\!-\!\!\bullet\,\ Q_{\rm Q}(f)$ ⇒ quantized source signal (from German: "quantisiert" ⇒ "Q")
$q_{\rm C}(t)\ \circ\!\!-\!\!\!-\!\!\!-\!\!\bullet\,\ Q_{\rm C}(f)$ ⇒ coded source signal (from German: "codiert" ⇒ "C"), binary
$s(t)\ \circ\!\!-\!\!\!-\!\!\!-\!\!\bullet\,\ S(f)$ ⇒ transmitted signal (from German: "Sendesignal"), digital
$n(t)$ ⇒ noise signal, characterized by the power-spectral density ${\it Φ}_n(f)$, analog $r(t)= s(t) \star h_{\rm K}(t) + n(t)$ ⇒ received signal, $h_{\rm K}(t)\ \circ\!\!-\!\!\!-\!\!\!-\!\!\bullet\,\ H_{\rm K}(f)$, analog
Note: Spectrum $R(f)$ can not be specified due to the stochastic component $n(t)$.
$v_{\rm C}(t)\ \circ\!\!-\!\!\!-\!\!\!-\!\!\bullet\,\ V_{\rm C}(f)$ ⇒ signal after decision, binary
$v_{\rm Q}(t)\ \circ\!\!-\!\!\!-\!\!\!-\!\!\bullet\,\ V_{\rm Q}(f)$ ⇒ signal after PCM decoding, $M$–level
Note: On the receiver side, there is no counterpart to "Quantization"
$v(t)\ \circ\!\!-\!\!\!-\!\!\!-\!\!\bullet\,\ V(f)$ ⇒ sink signal, analog
Here follows a short summary with regard to the use with ISDN. The graphic shows the block diagram of the PCM transmission system, which is adapted to the situation with ISDN. You can see:
- The analog (i.e.: value and time continuous) source signal $q(t)$ is converted into the binary signal $q_{\rm C}(t)$ by the three function blocks Sampling – Quantization – PCM Coding. In the graphic, this is shown in the upper signal path.
- The block with gray background shows the "digital transmission system" with transmitter, channel distortions and noise addition and the receiver, which among other things contains a decision. The channel output signal $v_{\rm C}(t)$ is binary like $q_{\rm C}(t)$.
- In the lower branch you can see the PCM decoder with the time-discrete, but now higher-level output signal $v_{\rm Q}(t)$. This is followed by the signal reconstruction to obtain the analog signal $v(t)$, for which an ideal rectangular low-pass filter is sufficient.
- There is no equivalent for the quantization at the receiver end. This means: The quantization errors that are unavoidable at the transmitter are irreversible. For this reason, $v(t) ≠ q(t)$ applies in principle to PCM as to any form of digital signal transmission.
- An important quantization parameter is the level number $M = 2^N$, where $N$ indicates the number of binary characters required for a sample.
- The larger $N$, the less the interfering influence of quantization and the higher the quality of the PCM system.
All these statements apply to PCM in general. Now the special features of pulse code modulation at ISDN are mentioned.
$\text{Pulse code modulation with ISDN:}$
The sampling in the time interval $T_{\rm A}$ takes place according to the "sampling theorem". This states:
- If the spectrum $Q(f)$ of the analog source signal $q(t)$ has components up to the frequency $f_{\rm NF, \hspace{0.1cm}max}$, the following must apply for the sampling rate:
- $$f_{\rm A} = \frac{1}{T_{\rm A} } \ge 2 \cdot f_{\rm NF, \hspace{0.05cm}max} \hspace{0.05cm}.$$
- ISDN telephone signals contain spectral components between $300 \ \rm Hz$ and $3400 \ \rm Hz$ and the sampling rate is $f_{\rm A} = 8 \ \rm kHz$ ⇒ $T_{\rm A} = 125 \ \rm µ s$. Thus, the sampling theorem is satisfied.
⇒ As mentioned before, quantization to $M$ possible signal values leads to irreversible errors. Because of the following binary PCM coding, a power of two is always chosen for $M$. Thus, each of the $M$–level input values can be represented by $N = \log_2 \hspace{0.05cm} (M)$ binary symbols ("bits"). In this dimensioning, it should be noted:
- The "quantization signal-to-noise power ratio" is $ρ_{\rm Q} ≈ M^2 = 2^{2N}$. This quantity describes the resulting SNR $ρ_v$ at the sink, assuming no additional transmission errors. When interference $($or noise$)$ is taken into account, the sink SNR $ρ_v$ is always smaller than the quantization SNR $ρ_{\rm Q}$.
- By using large values of $M$ or $N$, one can increase the PCM quality at the expense of the effort, the transmission rate, and the high-fruquency bandwidth thus required. For ISDN, a good compromise $($for the 1990s$)$ between desirable quality and required bit rate was standardized with $N = 8$ ⇒ $M = 256$.
- The ISDN bit rate $($for each of the two bearer channels$)$ is $8 · 8000 \ \rm 1/s = 64 \ kbit/s$ according to the above specifications. The quantization SNR is thus
- $$\rho_{\rm Q} = 2^{16}\hspace{0.3cm}\Rightarrow \hspace{0.3cm} 10 \cdot {\rm lg}\hspace{0.1cm}\rho_{\rm Q}= 10 \cdot {\rm lg}\hspace{0.1cm}2^{16}\approx 48\,{\rm dB} \hspace{0.05cm}.$$
- Compact disc $\rm (CD)$ quality $(N = 16 \ ⇒ \ M = 65\hspace{0.05cm}536)$ would result in $10 · \lg ρ_{\rm Q} ≈ 96 \ \rm dB$. However, this would require doubling the bit rate to $128 \ \rm kbit/s$.
Let us now look at the gray block in the PCM block diagram. In ISDN, the transmitter does not include a modulator for frequency conversion and the receiver does not include a demodulator. In other words: ISDN is a baseband transmission system with the following special features:
- In the ISDN transmission system, a redundant ternary transmitted signal $s(t)$ is used, with the "modified AMI code" on the $S_0$ interface $($"house connection"$)$ and "4B3T coding" on the $U_{\rm K0}$ interface $($"access network"$)$.
- The dominant noise $n(t)$ is the "near-end crosstalk" of adjacent line pairs. Many of the statements given in the book "Digital Signal Transmission" for AWGN noise are only conditionally valid for this type of noise.
Origin and historical development
In the following, some data on the historical development of digital transmission and switching technology – especially ISDN – are compiled. Here we restrict ourselves predominantly to the developments in Germany. Further information can be found in [Sie02][2].
- Around 1970 The need for digital subscriber lines is recognized worldwide; this is the beginning of digital transmission technology with pulse code modulation.
- 1979 Decision by Deutschen Bundespost $\rm (DBP)$ to digitize all exchanges.
- Around 1980 First ISDN specification by "Comité Consultatif International Téléphonique et Télégraphique" $\rm (CCITT)$ – today "International Telecommunication Union" $\rm (ITU)$.
- 1982 Decision of the DBP for the introduction of ISDN and concretization of the plans. However, it takes another seven years before it is introduced.
- 1984/85 The DBP puts the first digital long-distance and local exchanges into operation.
- 1987 Start of two German ISDN pilot projects of the DBP in Mannheim and Stuttgart.
- 1989 Official start of operation of national ISDN on March 8 at CeBIT in Hanover; specification of a uniform Europe-wide ISDN $($"Euro–ISDN"$)$.
- 1993/94 ISDN coverage in the old federal states of the Federal Republic of Germany; start of Deutsche Telekom's broadband ISDN pilot project $\rm (ATM)$.
- 1995 Official introduction of pan-European ISDN based on the "DSS1 standard" $($"Euro–ISDN"$)$.
- 1996 Introduction of the broadband ISDN bill service.
- 1998 Fully digitized network in Germany.
The left diagram of the following figure shows the increase of ISDN subscribers in Germany (blue bars).
- As early as 1999, the ten million mark is passed and by 2002, there are already 20 million ISDN subscribers in Germany.
- By 2004, half of all narrowband channels are already digital, after the number of analog telephone lines had already begun to decline significantly in 2000.
- However, the graph also shows a certain saturation (in mathematical terms: a negative second derivative) of the ISDN curve.
- This is directly related to the success story of $\rm DSL$ ("Digital Subscriber Line"), which began around 2001.
More on this in the "second main chapter" of this book.
Exercises for the chapter
Exercise 1.1: ISDN Supply Lines
References
- ↑ Pollakowski, M.; Wellhausen, H.W.: Eigenschaften symmetrischer Ortsanschlusskabel im Frequenzbereich bis 30 MHz. Mitteilung aus dem Forschungs- und Technologiezentrum der Deutschen Telekom AG, Darmstadt, Verlag für Wissenschaft und Leben Georg Heidecker, 1995.
- ↑ Siegmund, G.: Technik der Netze. 5. Auflage. Heidelberg: Hüthig, 2002.